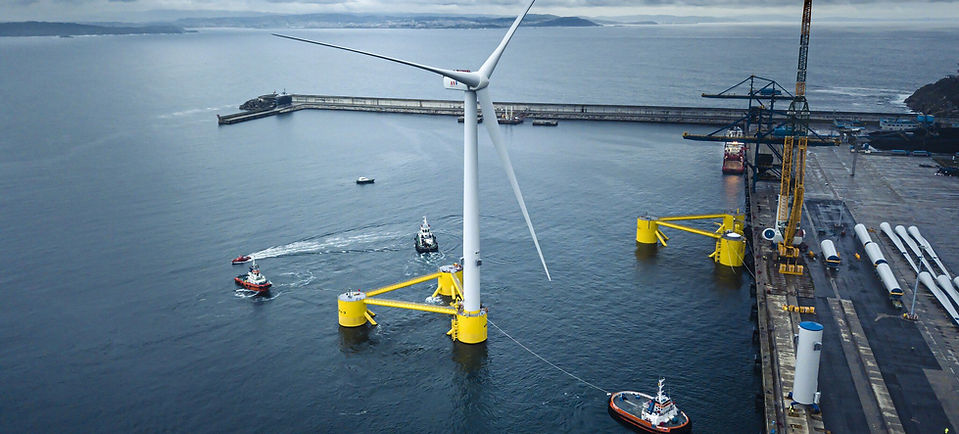
Beyond the Horizon: The Future of Offshore Wind is Floating
February 26, 2025
The global energy landscape is undergoing a dramatic transformation, driven by the urgent need to decarbonize our economies and mitigate the impacts of climate change. Offshore wind energy has emerged as a critical component of this transition, offering a clean, abundant, and increasingly cost-competitive alternative to fossil fuels. However, the full potential of offshore wind has been constrained by the limitations of traditional fixed-bottom installations, restricting development to shallower coastal waters. Floating offshore wind turbines represent the future of offshore power, unlocking access to vast, untapped wind resources in deeper waters and ushering in a new era of clean energy generation.
The Untapped Potential of the Deep
Offshore wind offers significant advantages over its onshore counterpart, including higher capacity factors due to stronger and more consistent winds. Traditional fixed-bottom offshore wind turbines, however, are economically and technically limited by water depth and complex seabed conditions. These limitations significantly restrict the geographic scope of development. Floating platforms, anchored to the seabed by flexible mooring systems, overcome these constraints, enabling turbines to be deployed in deeper waters where wind resources are significantly more abundant and consistent. Crucially, around 80% of the world's exploitable offshore wind resources reside in waters deeper than 60 meters (~200 ft.), a domain currently inaccessible to fixed-bottom installations. Floating offshore wind thus represent a critical pathway to harnessing this vast, untapped energy potential.
While the global floating wind industry remains in its early stages, with approximately 270 MW of operational capacity as of 2023, the future appears exceptionally promising. The global project pipeline has surged to 244 GW, demonstrating substantial industry momentum. The United States, recognizing its vast deep-water resources, currently has over 6 GW of floating projects in its development pipeline, with a significant portion under site control. Given that over two-thirds of the nation's offshore wind potential lies in deep waters, a 2022 study by the National Renewable Energy Laboratory (NREL) estimates the U.S. technical potential for floating offshore wind at a staggering 2,773 GW, capable of generating nearly 9,000 terawatt-hours of energy annually.
Technological Innovation at the Forefront
Floating offshore wind farms consist of wind turbines mounted on floating platforms, which are stabilized by sophisticated mooring and anchoring systems. Just like fixed-bottom offshore wind farms, the kinetic energy of the wind is captured by the turbine blades, converted into electricity, and transmitted via subsea cables to onshore substations for distribution. Several innovative platform designs are under development, each tailored to specific environmental conditions and project requirements:
Barge Platforms: Characterized by their large surface area in contact with the water, barge platforms offer inherent stability, similar to a ship. Their relatively simple design makes them a potentially cost-effective solution for certain applications.
Semi-submersible Platforms: These platforms minimize their exposure to wave action by reducing the water plane area while maximizing submerged volume for buoyancy. This design offers enhanced stability in challenging sea states.
Spar Platforms: Spar platforms achieve stability through a deep-draft design, with the majority of the weight concentrated at the lowest point. This approach provides excellent stability but can present challenges in manufacturing and deployment.
Tension Leg Platforms (TLPs): TLPs are anchored to the seabed using tensioned tendons, effectively minimizing platform motion. This design offers the potential for cost reduction by minimizing the size of the floating structure.
The selection of the optimal platform type is a complex decision, influenced by a multitude of factors including site-specific conditions, water depth, wind resource characteristics, turbine size, cost considerations, and supply chain availability.
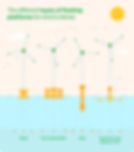
Mooring Systems
Mooring systems are essential for maintaining the stability and position of floating wind turbine foundations, especially in deep water. These systems, comprising mooring lines and anchors, transfer forces from the foundation to the seabed, counteracting unwanted motions that could damage subsea power cables. They are typically composed of various steel chain sections alternating with some sections composed of synthetic fiber rope, usually polyester or nylon. Mooring configurations are tailored to site conditions, foundation type, and cable design, influencing the turbine's six degrees of motion. Taut mooring lines, often used with tension leg platforms, connect the platform to high-load vertical anchors. Catenary lines, common in spar, barge, and semi-submersible platforms, utilize freely hanging chains and drag anchors.
Anchors
Anchors are critical for securing floating wind platforms to the seabed, and their design is heavily influenced by seabed characteristics. While various types exist, including deadweight, driven pile, drag, suction pile, gravity drop, and vertical load anchors, drag anchors are the most common due to their strong horizontal load resistance and good seabed penetration. However, they are less suited for vertical loads. Driven piles and suction piles offer alternative solutions, with suction piles also offering recoverability. A key innovation being explored is shared anchor systems, which allow multiple platforms to connect to a single anchor. This approach, demonstrated by Equinor's Hywind Tampen project, can reduce the total number of anchors required, improving efficiency and potentially lowering costs compared to projects like Hywind Scotland.
More information on anchors and moorings: Fact sheet from offshore wind Scotland
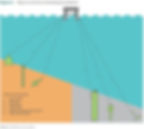
Transmission Cables
A key element for floating offshore wind cabling is the fact the cables are dynamic, meaning that they are designed to follow and withstand the motion of the floating sub-structure caused by wind, waves and current. They are developed specifically to be exposed to saltwater, to have high fatigue loads and to have tolerance to the motions of foundations and oceans. Dynamic cables usually have a non-lead insulator sheath and an additional armoring layer when compared to static cables.
The Multifaceted Advantages of Floating Wind
The adoption of floating offshore wind technology offers a compelling array of benefits:
Access to Superior Wind Resources: Floating turbines unlock access to stronger, more consistent winds further offshore, resulting in significantly higher capacity factors compared to fixed-bottom installations. Capacity factors exceeding 60% are achievable, representing a substantial improvement over traditional fixed-bottom projects.
Reduced Environmental Footprint: By locating further from shore, floating offshore wind farms minimize impacts on sensitive coastal ecosystems and marine life. Less noisy installation methods, such as the use of drag anchors and suction piles, further reduce disturbance to marine animals.
Streamlined Manufacturing and Deployment: Floating platforms can be constructed and assembled onshore, simplifying logistics and reducing reliance on expensive heavy-lift vessels. Towing the completed platforms to the offshore site minimizes weather-dependent operations and facilitates easier maintenance, with some operations potentially conducted in port.
Enhanced Public Opinion: The greater distance from shore reduces the visual impact and noise associated with wind farms, minimizing potential community resistance which can help facilitate smoother project development.
Driving Cost Competitiveness: The floating offshore wind industry is experiencing rapid cost reductions, driven by technological advancements, economies of scale, and optimized manufacturing and installation processes.
Stimulating Local Economies: Onshore assembly and manufacturing foster the development of local supply chains, creating valuable jobs and stimulating economic growth in coastal communities. The development of dedicated port infrastructure further enhances these economic benefits.
Enhanced Scalability and Standardization: The potential for standardized platform designs offers significant cost advantages and accelerates deployment, enabling the rapid scaling of floating wind capacity.
Navigating the Challenges
While floating offshore wind holds immense promise, its widespread adoption faces a complex web of challenges that must be addressed to unlock its full potential. These challenges span technical, cost, environmental, regulatory, and infrastructural domains.
Technical Challenges
Deep Water Installation: Deploying massive wind turbines in the challenging environment of deep ocean waters presents significant logistical hurdles. Specialized vessels capable of handling and installing these large structures in deep water are required, driving up costs and demanding innovative installation techniques.
Mooring Systems: The heart of a floating wind farm lies in its mooring system. Designing robust and reliable mooring systems that can withstand extreme weather conditions, including high winds, strong currents, and large waves, is crucial for maintaining platform stability and ensuring long-term operational integrity.
Weather Dependence: Installation and maintenance operations for floating wind farms are inherently dependent on favorable weather windows. Rough seas and high winds can significantly disrupt these activities, leading to delays and increased costs. Developing strategies to mitigate weather-related risks is essential.
Cable Management: Managing the intricate network of underwater cables that connect the floating turbines to the onshore grid poses a significant technical challenge. Protecting these cables from damage caused by marine life, strong currents, and other environmental factors is vital for reliable energy transmission. Transmitting electricity over longer distances can also result in greater efficiency losses, which can reduce the overall output to the grid.
Cost Challenges
High Capital Investment: The specialized technology required for floating wind farms, including the sophisticated floating foundations, advanced mooring systems, and subsea cables, necessitates substantial upfront capital investment. Reducing these initial costs is crucial for making floating wind competitive with other energy sources.
Operation and Maintenance: The remote location of floating wind farms, often far offshore, makes operation and maintenance activities complex and expensive. Developing cost-effective strategies for accessing turbines for repairs and maintenance, particularly in harsh weather conditions, is essential for long-term economic viability.
Environmental Challenges
Marine Life Impacts: The construction and operation of floating wind farms have the potential to impact marine ecosystems. Noise from construction activities, electromagnetic fields from subsea cables, and the presence of turbine structures can potentially disrupt fish migration patterns, marine mammal behavior, and other aspects of the marine environment. Careful environmental assessments and mitigation measures are essential to building these projects in a responsible manner..
Regulatory Challenges
Permitting Complexities: Navigating the complex and often lengthy permitting processes associated with offshore wind development can be a significant hurdle. Streamlining these processes while ensuring environmental protection is crucial for accelerating project timelines.
Grid Connection: Integrating the electricity generated by floating wind farms into the existing power grid requires careful planning and coordination. Upgrading grid infrastructure and ensuring grid stability are essential for accommodating large-scale floating wind deployment.
Infrastructure Challenges
Port Limitations: The construction and assembly of large floating wind turbines require specialized port facilities with sufficient capacity, heavy-lift capabilities, and deep-water access. Many existing ports lack these capabilities, requiring significant investment in port infrastructure development.
Vessel Availability: The installation and maintenance of floating wind farms require specialized vessels capable of operating in deep water and harsh weather conditions. The limited availability of these vessels can create bottlenecks and increase costs.
Addressing these multifaceted challenges requires a concerted effort from industry, government, and research institutions. Continued innovation in technology, streamlined regulatory processes, strategic infrastructure investments, and a commitment to environmental stewardship are crucial for realizing the full potential of floating offshore wind and powering a sustainable future.
Operational Developments
Several floating offshore wind projects have demonstrated the viability and potential of this technology. Hywind Scotland, the world's first floating wind farm (30MW), has consistently achieved the highest average capacity factor of all UK offshore wind farms for three years running (reaching 57.1% in 2020), proving the potential of floating wind. Equinor, the developer, has achieved significant cost reductions (60-70%) between its demonstrator project and Hywind Scotland and anticipates further reductions (40%) with its larger 88 MW Hywind Tampen project. Hywind Tampen, the world's largest floating wind farm, powers offshore oil and gas platforms and serves as a testbed for future floating wind technologies. These projects showcase the technical feasibility, increasing cost-competitiveness, and real-world performance of floating offshore wind, paving the way for larger-scale deployments.
Check out this video by Equinor about Hywind Scotland, the worlds first floating offshore wind project.
Other Pilot Projects
-The 25-MW WindFloat Atlantic project: The first floating wind farm in continental Europe, features three 8.4 MW turbines utilizing semi-submersible platforms. It has been operational since 2019, supplying clean energy to the 25,000 Portuguese households every year
-The 25-MW Provence Grand Large pilot project: Three 8.4-MW Siemens Gamesa turbines on tension-leg floating platforms near Marseille, France. It is expected to produce the equivalent of the annual electricity consumption of 45,000 inhabitants.
-The 3.6-MW Guoneng Sharing pilot project: A single turbine on a semisubmersible platform near Longyuan Nanri Island in China.
-The 2-MW DemoSATH demonstration project in Spain: A single 2-MW turbine, designed to test the "SATH" (Saitec Offshore Technologies Hull) floating platform technology in real-world conditions off the Basque coast.
While most other projects are still in the planning phase, it is estimated that around 14 GW of floating offshore wind capacity will be installed globally by 2029. Still, there is a high degree of uncertainty about their timing and likelihood of completion. Most of the developer announced deployment through 2029 is in the United Kingdom (4,242 MW), Italy (4,160 MW), Taiwan (1,530 MW), China (1,052 MW), and Spain (995 MW).
The First Two-Turbine Floating Platform
Mingyang Smart Energy has launched OceanX, a groundbreaking floating offshore wind platform featuring two 8.3MW turbines for a combined capacity of 16.6MW, making it the world's largest single-capacity floating wind turbine platform. Designed to withstand Category 5 hurricane conditions and continue generating power in winds up to 161 mph and waves as high as 98 feet, OceanX is expected to produce enough electricity to power approximately 30,000 Chinese households annually. A 1:10 scale prototype was successfully tested in 2020, and the full-scale platform has now been deployed to the Qingzhou IV offshore wind farm in Yangjiang, Guangdong, China. This innovative dual-turbine design, built with ultra-high-performance concrete and featuring 219-meter towers, represents a significant advancement in floating offshore wind technology.
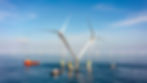
Charting the Course for a Sustainable Future
Floating offshore wind is not merely a promising technology; it is a transformative force poised to reshape the global energy landscape. By unlocking access to previously inaccessible wind resources, floating offshore wind farms have the potential to become a cornerstone of the clean energy transition. While challenges remain, the industry is rapidly maturing, propelled by innovation, investment, and a growing recognition of the immense potential of this technology. With continued focus on supply chain development, port infrastructure, and O&M strategies, floating offshore wind is poised to play a leading role in powering a sustainable future. Innovation in floating offshore wind technology is the key to unlocking the vast, untapped energy potential of deeper waters, paving the way for a cleaner and more secure future.
Sources
Equinor, NREL, OSW Biz, Iberdrola, Semar, Science Direct, Acteon, IRENA